Opioid receptors are the primary way alkaloids from Mitragyna speciosa (kratom) and other opiates affect the body. Their primary purpose is to maintain a neurological balance (homeostasis) after pain sensations, but they also influence several different functions.
Opioid receptors use and interact with several elements of the brain and body, keeping them in two-way communication at all times.
Here are the elements we’ll cover with links to their sections if you want to jump ahead:
- The Basic Function of Opioid Receptors
- How the Body Feels Pain
- How Opioids Regulate Pain
- Opioid Receptor Activation — Stopping the receptor cell’s activity and interrupting the communication flow in the pain pathway.
- Cellular Alterations After Activation — We’ll break down the molecular changes receptor activation sets in motion.
- Strategic Placement — How the physical location of opioid receptors plays a significant role in their function.
- Functions of Different Receptors
- Mu-Opioid Receptors — The primary receptors that kratom and other opioids interact with.
- Delta-Opioid Receptors — Potentially a future site of action for anti-inflammatory drugs
- Kappa-Opioid Receptors — The “psychedelic” receptor responsible for the effects of Salvia divinorum’s main active alkaloid, salvinorin A.
- Nociceptin Receptors — Late to the research game, these receptors are promising yet poorly understood.
- How Kratom Interacts With Opioid Receptors
- How Mitragynine and 7-Hydroxymitragynine Influence Opioid Receptors
- How Other Kratom Alkaloids Influence Opioid Receptors
- The Potential for Medicalizing Kratom Alkaloids
- Mastering and Respecting Your Body’s Neurochemistry
While kratom may bind to dopaminergic, serotoninergic, and other receptor systems, the “pain pathway” it operates on is by far the most important.
Let’s dive into the body and explore our protective and restorative mechanisms as they’re best exemplified — through the opioid receptors.
How Opioid Receptors Function
Receptors communicate with the cell they’re attached to and impart messages from outside chemicals to instruct different physiological changes. These interactions usually alter chemicals that produce, store, communicate, restrict, and empower critical functions.
Opioid receptors are members of the crucial Seven transmembrane g-coupled protein receptors (7TMR) class, which contain a bound protein encoded for specific functions. When resting in an inactive state, they exchange messages back and forth with the brain about what the body needs.
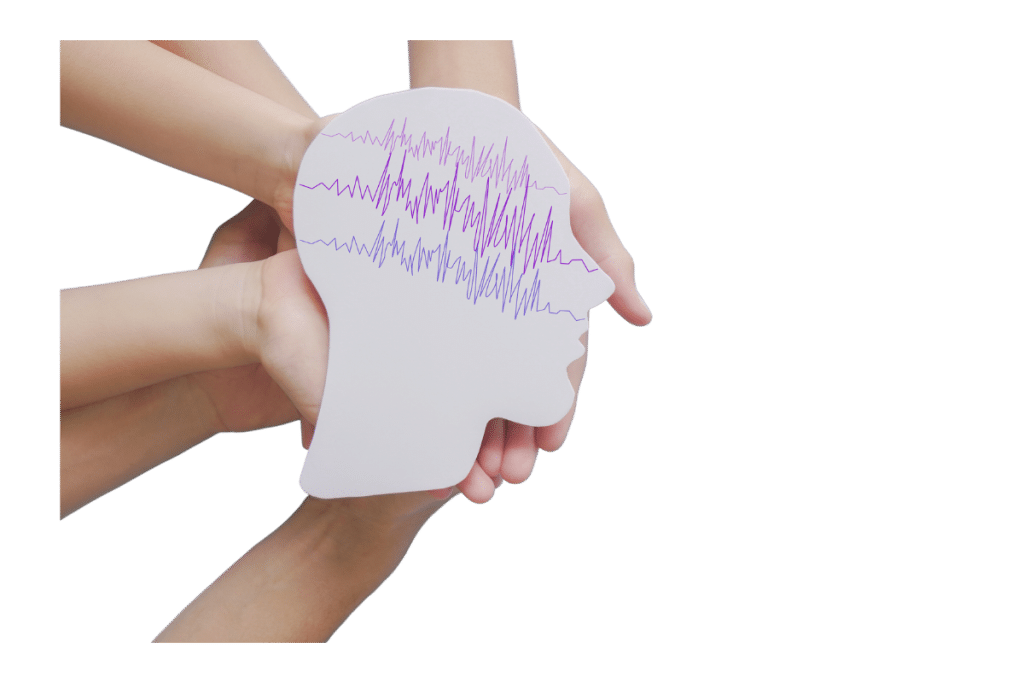
Our opioid system regulates several functions, but the main one is pain. After experiencing pain, your body sends a distress signal to the brain and triggers the release of endogenous opioids.
Activation of the opioid receptor’s main result is cutting off the communication of pain signals. As such, it’s essential to discuss the physiological effects of pain before diving into how opiates treat it.
How Does the Body Feel Pain?
Pain results from a cascade of neurological signals to and from our brain, depending on where the pain originates and how long you’ve been experiencing it [1]. In this way, it is “all in the mind,” though not in the dismissive sense — literally everything we perceive happens there.
Here’s how the brain responds to pain in the hypothetical situation of spraining an ankle:
- Irritation and inflammation stimulate nociceptors — receptors responsible for communicating pain.
- As they build, they travel to the dorsal horn — located inside the ridge on the backside of your spine.
- This is an “information superhighway,” shooting the message straight to the thalamus — a brain region that distributes it as needed.
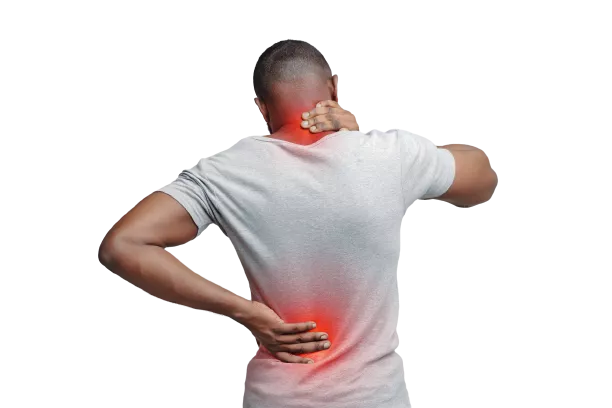
As you may be aware, pain persists long beyond the initial shock of the injury. Even after swelling recedes and sensitivity to touch decreases, you may still feel pain.
This is thanks to our brain communicating that we still need to take it easy as we heal, or there may be underlying pain we aren’t aware of. On occasion, this message may extend beyond this and result in psychosomatic pain — a perception of pain despite the absence of injury.
If you didn’t continue feeling pain in your ankle after the event, you might partake in a risky activity and make it far worse without knowing. Through this communication — or the “descending pathway” of pain regulation — your brain can assist in healing and awareness.
How Opiates Regulate Pain
Opiates cut off the flow of communication, blocking the pain and discomfort you’re supposed to feel. Whether natural (endogenous) or pharmacological (exogenous), opioid receptor activation has the same goal.
Broadly speaking, here’s how opioid receptors interact with their activating chemical messengers [2]:
- A molecule binds to the receptor, activating it.
- Activation causes two proteins attached underneath to decouple and release from their docked position underneath the receptor.
- After dissociating, they individually signal a series of changes within the cell.
- Together, the changes increase the capability of a cell while reducing its potential to create chemical messengers responsible for pain.
- Some of these receptors are near other critical areas in the brain and influence changes in, among other things, mood and feelings of pleasure through dopamine and serotonin.
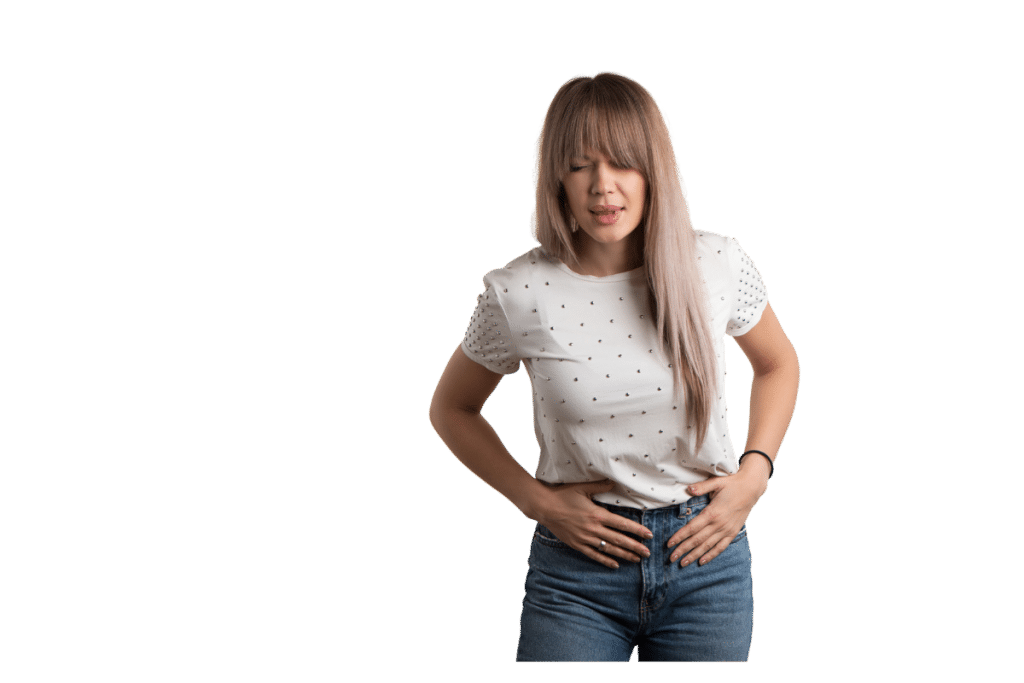
When you burn your hand on a hot stove, your opioid system tells your brain to pull back and reminds you of your poor choices for weeks to come. However, you’ll probably feel nothing immediately after the fact — as though the pain is waiting to “kick in.”
This is because, when you take an opiate — or release them endogenously — it feels as though the pain leaves entirely. Medications may take time to kick in, but your body’s messages apply an analgesic (pain-relieving) effect immediately.
This is your opioid system blocking you from the trauma of the injury. In reality, the pain is still there; your body is simply not telling you about it. It’s giving you time to process what happened before the pain sets in.
Cellular Alterations from Opioid Receptor Activation
7TMRs, like those in the opioid system, are vital components of regulating our physiological function. After activation, they release two attached proteins with specific tasks of cellular alteration.
Here are a few of the changes they are responsible for initiating [2, 3, 4]:
- Increasing the production of positively-charged potassium ions (K+) and releasing them across neurons. This results in the cells shutting down from sending and receiving signals — or polarization.
- One subunit binds directly to the channel for calcium ions — responsible for carrying pain signals, among other things — blocking the point of entry. It may do so after initiating some of the other changes, but we aren’t entirely sure which protein does what outside of this.
- Cyclic adenosine monophosphate (cAMP), which helps deliver messages deeper within the cellular system, depends on reactions from calcium to occur. As such, calcium restriction also inhibits it.
- Adding a phosphate group — or phosphorylation — to the cell enables it to capture long molecules called β-arrestins, preventing them from doing their duty of reversing the effects.
These are just the most important cellular alterations, and several others are responsible for the analgesic and other effects. Additionally, the location of opioid receptors often means they are inherently communicating with other systems within the brain and body.
Compounds capable of triggering this entire process are full-agonists — including the natural compound morphine and its synthetic derivatives.
Importantly, the final two points also contribute to respiratory depression, the most dangerous side-effect of opiates. Since cAMP and β-arrestins assist in regulating airways and lung function, inhibiting their production and trapping them (respectively) can result in shallow breathing or even stopping the process altogether.
Opioid Inhibitory Locations
We’ll step back from the molecular side of opioid’s pharmacological effect because it’s important to note where it’s primarily active. In addition to occupying critical locations for pain communication, they’re often close to other essential areas of the brain and may activate or deactivate them by proxy.
When it comes to the pain pathway, the main areas where opioids are active include:
- The skin and other peripheral tissue (potentially)
- In the dorsal horn and spinal cord
- Throughout the brain — including the medulla, which sits at the base of the brainstem and is vital for relaying messages to the rest of the body.
These locations represent the three biggest points of the pain pathway and allow it to restrict the flow easily. Whether messages originate at the location of the injury, have made it to the spinal cord, or are coming directly from the brain, opiates cut them off.
Additionally, many of the receptors are near the dopaminergic and serotonergic systems and can stimulate or suppress their production. Theoretically, its relation to dopamine is instrumental in dependence formation, releasing the rewarding chemical in the brain and reinforcing drug use.
However, some opioid receptors suppress dopamine production or don’t influence it at all.
Different Opioid Receptors & What They Do
The three main opioid receptors within the body are kappa- (κ,) delta- (δ,) and mu- (μ) opioid receptors. In addition to this is the little-known nociceptin receptor, which, though related, doesn’t seem to interact with typical opioid agonists or antagonists.
Broadly speaking, all three have the same function, but their location changes the result of what this accomplishes. Opiates in medical settings often focus on the mu-opioid receptor (MOR), which exerts the most considerable pain-relieving effect.
Exogenous opioids like morphine, heroin, kratom, and others likely influence the three main receptors in some way. However, the different actions of each have led some researchers to consider the benefits of targeting them specifically.
Activation of the kappa- and delta-opioid receptors (KOR/DOR) has very different effects from the MOR — often including a lower risk of forming a dependence and experiencing side effects.
Let’s take a look at each of the opioid receptors and the different activities they exhibit:
What Do Mu-Opioid Receptors Do?
MOR activation brings on the most potent analgesic effect, so it’s frequently the target of opiates. They’re located in crucial positions throughout the pain pathway and also influence our feelings of reward and euphoria [5], triggering dopamine and serotonin release to accomplish this.
These chemicals are foundational to human life and how we experience our existence. Together, they form significant parts of our body’s reward system, providing bursts of energy and happiness to reinforce behaviors it enjoys — both healthy and unhealthy.
It also helps improve a person’s mood and calm people down when they’re experiencing pain or discomfort. Together with the relaxing effects of the opioid system, it’s easy to see how this contributes to addiction to synthetic opioids.
However, it also serves a vital role in pain management by providing an energizing boost to mood when someone needs it most.
Another system MORs affect is the digestive system, where they help relax and constrict muscles responsible for the process. As a result, constipation and gastrointestinal distress are common.
It’s also worth noting that the high potency of MOR-targeting has a downside. Along with higher strength comes an increase in side effects like respiratory depression.
What Do Kappa-Opioid Receptors Do?
KOR agonists inhibit the release of dopamine instead of releasing it, helping to regulate mood in times of elevated stress after an injury. More potent activators of this receptor can also induce a psychedelic effect. Salvinorin A, the hallucinogenic compound from Salvia divinorum, is a great example [6].
KOR’s proximity to production areas of dopamine and its receptors antagonizes the release and absorption of dopamine [7]. Dynorphin — the endogenous chemical for this receptor — usually releases after prolonged exposure to pain, such as high heat levels or heavy exercise.
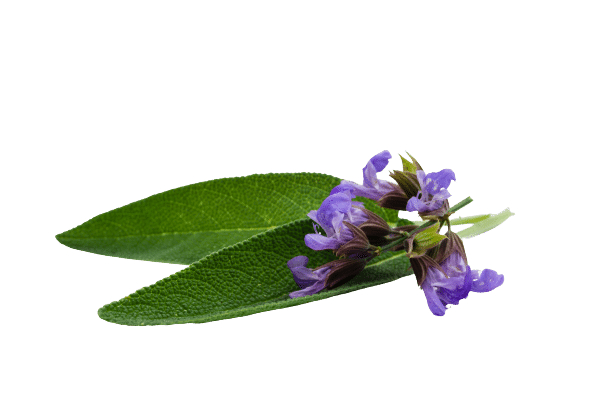
This may help mediate the high levels of dopamine, which are likely there to combat the anxiety of your experience, as high dopamine levels can trigger anxiety and irritation. Its different mode of action leads some to consider it a less-addictive target for pain relief, with a lower risk of abuse.
However, medical options often swing to the other extreme; full agonists often lead to dysphoria — a general state of dissatisfaction or depression. Until we know how to partially agonize KOR without causing the side effects, it’s not likely a good option for treating pain.
Salvinorin A is interesting as it exclusively targets the KOR, interacting very little with any other systems (that we know of). The result is a dissociative and psychedelic state, effectively removing the communication signals for all sensations to and from the brain.
Despite its potent action, salvinorin A doesn’t seem to lead to depressive or dysphoric states, perhaps due to its short timespan (around 15 minutes).
What Do Delta-Opioid Receptors Do?
DORs receive little attention in the scientific community but offer some interesting potential for treatments [8]. While the immediate pain-relieving effect from activating DORs is rather weak, its location may make it useful for chronic and psychosomatic pain [9].
DORs often lay dormant within the cell, perhaps even “locked away” under normal conditions. Inflammation brings these receptors to the surface so they can receive signals and exert their effects.
If MORs are for extreme pain and KORs are for ongoing pain, DORs can be thought of as useful for the dull pain of inflamed joints or something similar. In fact, some research suggests DORs could be great tools for dealing with them.
Particularly, they may be helpful for topical applications or patches that can target the receptor directly at the site of inflammation.
Enkephalinur is our endogenous signaling molecule for this receptor and has the highest affinity for DORs [10]. While we don’t yet understand the full effects or function of how this system works or interacts with opiates, its effect on dopamine and serotonin is likely similar to MORs.
One study involving mice found that the antagonization of DORs in mice elevated fear and anxiety responses. This would resolve after giving them an agonist to reverse the effects, showing the vital role this system plays in emotion regulation [11].
Whether our systems work the same as those of mice is a question we have yet to answer.
What Do Nociceptin Receptors Do?
While each receptor pairs with a higher affinity for its unique peptide, most have some influence over the others — but not nociceptin. It’s similar to other peptides, but it doesn’t activate any receptor other than the nociceptin opioid peptide receptors (NOPRs) [12].
It was a breakthrough discovery in 1995 for the opioid system, subsequently becoming the name for the receptor. Before this, its name was “orphanin FQ,” since they didn’t know what “child molecule” might pair with it.
NOPR activation likely reverses other receptors’ pain-relieving and rewarding effects, interacting in ways we don’t yet fully understand. They may also be crucial for regulating mental health concerns like anxiety and depression along with learning capacity.
Nociceptin receptors’ location in key areas of dopamine production and release seems to enable this. In one study, researchers gave rats cocaine and then injected them with nociceptin. They found it mediated the increased dopamine that cocaine usually provided [13].
Though new to the game, there’s no shortage of intrigue with NOPRs, and their potential is very bright.
How Does Kratom Interact With Opioid Receptors?
Kratom (Mitragyna speciosa) interacts with several opioid receptors in varying capacities [14], but we’re not sure how quite yet. Researchers have been looking into the question for a long time, but we still don’t have definitive evidence.
The diverse range of opiates it contains, and the range of effects they produce, are likely a major component of its overall activity and contribute to the complications of understanding it. While kratom often helps with pain, it’s also a stimulant with varying effects, depending on the strain and dose.
This points to a complex pharmacology with various factors. We know of over 40 alkaloids within the kratom plant and a handful that have shown activity with opioid receptors.
The top four are:
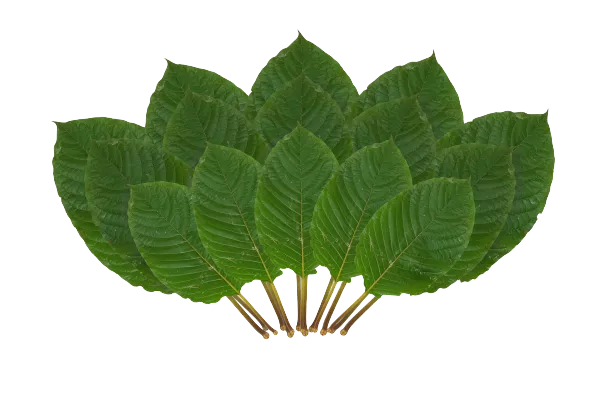
Unlike synthetic opioid medications, kratom compounds are partial agonists, meaning they only partially activate the receptor. In this case, they signal the proteins to cut off pain communication but don’t recruit β-arrestins, which are a huge component of respiratory depression and other side effects.
Despite considerable effort, we have yet to fully understand how they interact with the various opioid receptors. Evidence suggests MOR, DOR, and KOR all receive some influence from kratom alkaloids — though KOR affinity is likely negligible.
Depending on the alkaloid and receptor in question, it may act in agonistic or antagonistic ways on receptors, contributing to its unique effect. Kratom’s largest impact comes from mitragynine, along with its active metabolite.
Related: Is Kratom An Opioid?
Effects of Mitragynine & 7-Hydroxymitragynine On the Opioid System
Compared to the gold standard of morphine, 7-hydroxymitragynine may be as much as 13 times as active [15]. Mitragynine is the more prominent compound in the plant but makes up as much as 66% of the plant’s alkaloid content.
While mitragynine is less potent, 7-hydroxymitragynine is one of its metabolites — meaning the former produces the latter after digestive enzymes begin breaking it down [16].
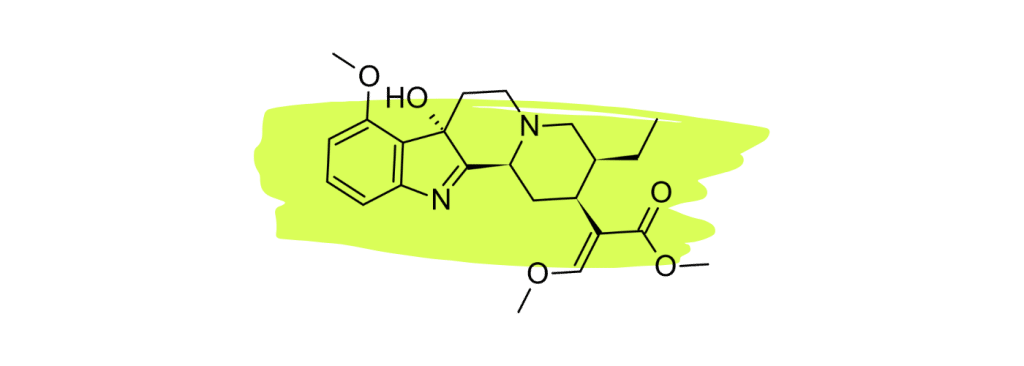
Both are partial agonists of the mu-opioid receptor and antagonists of the kappa- and delta-opioid receptors — with only a slight affinity for the latter [17]. This may point to mitragynine initially activating the receptors, followed by a stronger effect after the rest has a chance to pass through the liver.
Mu-opioid receptor activation is opioids’ primary mechanism for pain relief, so it makes sense this would be the principal mechanism of action. Throw in KOR antagonization to prevent dopamine restriction, and mitragynine exerts an anxiolytic (anti-anxiety) and antidepressant effect.
An essential element of 7-OH’s role as a metabolite revolves around the previous history of research into kratom involving animal models. Since the liver varies so drastically across species — even differences among individuals can be great — much of the prior research may be in question.
Introductory research often involves animal studies, so much of the foundation of what we know may be inaccurate if digestive systems play a role in the activity.
Related: Is Kratom Addictive?
Effects of Other Kratom Alkaloids On the Opioid System
Unlike natural medicine, pharmaceutical options usually only involve one or two active ingredients. Kratom, on the other hand, has over 40 alkaloids in its leaves — some active, some not, and others we have no understanding of.
Search our website for “alkaloid,” and you’ll see numerous worth reading about. Each one exhibits an impact and may counteract or empower others around it.
Research is scarce when it comes to any alkaloids from kratom outside of the main two, but scientists are taking note of their unique properties. While they may only occur in trace amounts within the plant or only appear in certain strains, we can isolate, synthesize, and study them.
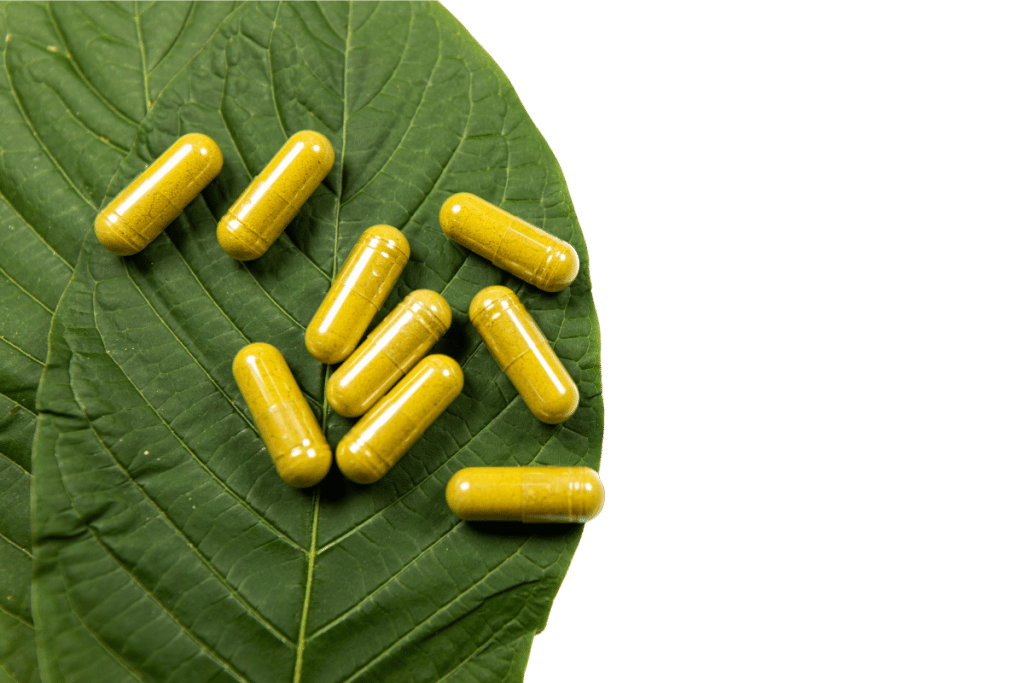
While we know only a handful of active opiate alkaloids (with mitragynine being most abundant by a long shot), there may still be more. We don’t yet understand what the compounds we know of are doing, let alone the others we have yet to research and test.
As such, kratom is an ever-evolving field of research into opiates, the receptors they communicate with, and pharmacological solutions for the future.
The Future of Kratom & Opioid Receptors
Kratom uniquely interacts with opioid receptors as a partial agonist for MOR to exert specific actions while restricting others. While MOR has the best potential for pain relief, kratom alkaloids also influence KOR and DOR in fascinating ways with promising pathways for research.
Researchers are looking at what we could learn from these natural alkaloids and harness their power. Morphine and the synthetic opioids we’ve created from it are good examples of how we have done this in the past with Papaver somniferum (the opium poppy plant).
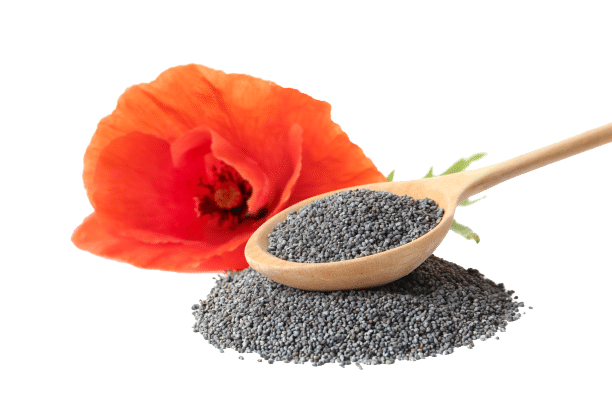
After discovering the pain-relieving powers of morphine from the sap of the poppy’s seed pods, we refined it into opium. This concentrated wax of morphine and other opiates was refined further for pharmaceutical-grade heroin, fentanyl, and others.
What if we were to go down this same line of refinement with the natural elements we find in kratom?
In 2017, researchers created “an oxidative rearrangement product” of corynantheidine along with mitragynine [18]. They found them to “show promise as potent analgesics with a mechanism of action that includes [MOR] agonism/[DOR] antagonism.”
They went on to note that it resulted in a slower build-up of tolerance, a lower likelihood of forming dependence, and fewer of the physiological side effects of morphine. Hopefully, this is the start of a tidal wave of research into what is possible through kratom.
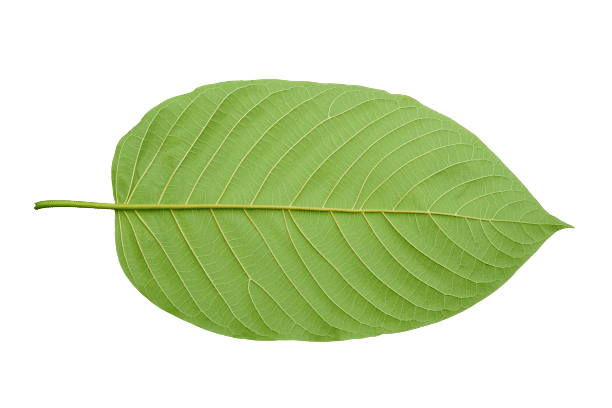
Some research suggests mitragynine is more dangerous than morphine — albeit in Zebrafish embryos [19] — but we know very little about it. Also, centuries of historic use make this seem unlikely.
Still, the capability to selectively agonize and antagonize various opiate receptors presents a bright path for research. Not to mention the activities of minor alkaloids within kratom and their potential.
Final Thoughts: Mastering & Tinking With Our Physiology
Our opioid system — like most others in the body — exists as a form of regulation. When something falls out of balance, it’s how your brain returns you to normal.
Unfortunately, our brain is connected to us, and we have yet to master the entirety of our existence. Additionally, sometimes our physiology falls short of providing everything we need from it, and historically, nature has always provided us with the solution.
Luckily, we have evolved alongside the tools to communicate with it and help it out through outside sources like kratom or other opiates.
We can’t produce our own nutrients or hydrate ourselves, so we have food and water. When we can’t quite build up the energy we need or focus on the task at hand, turning to plants or medications follows in the same vein.
Toying with our physiological makeup is a hallmark of the human experience, and we’ve been doing it since the dawn of time. Whether it’s cannabis, tobacco, coffee, or kratom, humans have turned to substances for assistance since (likely) before we evolved into humans.
When we do, it’s important to keep the original intention of our regulatory functions in mind: balance.
Our opioid receptors work hard to achieve homeostasis on their own, and a boost may give them a helping hand. Without regulation, however, this becomes their way of existence — their new normal is now abundance, so the goal of the regulatory system becomes reduction.
This may even lead to a total tolerance to opiates, cutting the system off entirely from their effects, regardless of dose. Even natural substances like kratom can build tolerance or lead to addiction.
Just remember: opiates and other drugs are here to guide the journey of our lives, not drive the car.
- Maldonado, R., Baños, J. E., & Cabañero, D. (2018). Usefulness of knockout mice to clarify the role of the opioid system in chronic pain. British Journal of Pharmacology, 175(14), 2791–2808. https://doi.org/10.1111/bph.14088
- Al-Hasani, R., & Bruchas, M. R. (2011). Molecular Mechanisms of Opioid Receptor-Dependent Signaling and Behavior. Anesthesiology, 115(6), 1363–1381. https://doi.org/10.1097/ALN.0b013e318238bba6
- Mores, K. L., Cassell, R. J., & van Rijn, R. M. (2019). Arrestin recruitment and signaling by G protein-coupled receptor heteromers. Neuropharmacology, 152, 15–21. https://doi.org/10.1016/j.neuropharm.2018.11.010
- Pathan, H., & Williams, J. (2012). Basic opioid pharmacology: An update. British Journal of Pain, 6(1), 11–16. https://doi.org/10.1177/2049463712438493
- Kosten, T. R., & George, T. P. (2002). The neurobiology of opioid dependence: Implications for treatment. Science & Practice Perspectives, 1(1), 13–20. https://doi.org/10.1151/spp021113
- Butelman, E. R., & Kreek, M. J. (2015). Salvinorin A, a kappa-opioid receptor agonist hallucinogen: Pharmacology and potential template for novel pharmacotherapeutic agents in neuropsychiatric disorders. Frontiers in Pharmacology, 6, 190. https://doi.org/10.3389/fphar.2015.00190
- Aldrich, J. V., & McLaughlin, J. P. (2009). Peptide Kappa Opioid Receptor Ligands: Potential for Drug Development. The AAPS Journal, 11(2), 312–322. https://doi.org/10.1208/s12248-009-9105-4
- Pradhan, A. A., Befort, K., Nozaki, C., Gavériaux-Ruff, C., & Kieffer, B. L. (2011). The delta opioid receptor: An evolving target for the treatment of brain disorders. Trends in Pharmacological Sciences, 32(10), 581–590. https://doi.org/10.1016/j.tips.2011.06.008
- Vanderah, T. W. (2010). Delta and kappa opioid receptors as suitable drug targets for pain. The Clinical Journal of Pain, 26 Suppl 10, S10-15. https://doi.org/10.1097/AJP.0b013e3181c49e3a
- Cullen, J. M., & Cascella, M. (2023). Physiology, Enkephalin. In StatPearls. StatPearls Publishing. http://www.ncbi.nlm.nih.gov/books/NBK557764/
- Szklarczyk, K., Korostynski, M., Cieslak, P. E., Wawrzczak-Bargiela, A., & Przewlocki, R. (2015). Opioid-dependent regulation of high and low fear responses in two inbred mouse strains. Behavioural Brain Research, 292, 95–101. https://doi.org/10.1016/j.bbr.2015.06.001
- Ubaldi, M., Cannella, N., Borruto, A. M., Petrella, M., Micioni Di Bonaventura, M. V., Soverchia, L., Stopponi, S., Weiss, F., Cifani, C., & Ciccocioppo, R. (2021). Role of Nociceptin/Orphanin FQ-NOP Receptor System in the Regulation of Stress-Related Disorders. International Journal of Molecular Sciences, 22(23), 12956. https://doi.org/10.3390/ijms222312956
- Vazquez-DeRose, J., Stauber, G., Khroyan, T. V., Xie, X. (Simon), Zaveri, N. T., & Toll, L. (2013). Retrodialysis of N/OFQ into the nucleus accumbens shell blocks cocaine-induced increases in extracellular dopamine and locomotor activity. European Journal of Pharmacology, 699(1–3), 200–206. https://doi.org/10.1016/j.ejphar.2012.11.050
- Eastlack, S. C., Cornett, E. M., & Kaye, A. D. (2020). Kratom—Pharmacology, Clinical Implications, and Outlook: A Comprehensive Review. Pain and Therapy, 9(1), 55–69. https://doi.org/10.1007/s40122-020-00151-x
- Halpenny, G. M. (2017). Mitragyna speciosa: Balancing Potential Medical Benefits and Abuse. ACS Medicinal Chemistry Letters, 8(9), 897–899. https://doi.org/10.1021/acsmedchemlett.7b00298
- Kruegel, A. C., Uprety, R., Grinnell, S. G., Langreck, C., Pekarskaya, E. A., Le Rouzic, V., Ansonoff, M., Gassaway, M. M., Pintar, J. E., Pasternak, G. W., Javitch, J. A., Majumdar, S., & Sames, D. (2019). 7-Hydroxymitragynine Is an Active Metabolite of Mitragynine and a Key Mediator of Its Analgesic Effects. ACS Central Science, 5(6), 992–1001. https://doi.org/10.1021/acscentsci.9b00141
- Kruegel, A. C., Gassaway, M. M., Kapoor, A., Váradi, A., Majumdar, S., Filizola, M., Javitch, J. A., & Sames, D. (2016). Synthetic and Receptor Signaling Explorations of the Mitragyna Alkaloids: Mitragynine as an Atypical Molecular Framework for Opioid Receptor Modulators. Journal of the American Chemical Society, 138(21), 6754–6764. https://doi.org/10.1021/jacs.6b00360
- Váradi, A., Marrone, G. F., Palmer, T. C., Narayan, A., Szabó, M. R., Le Rouzic, V., Grinnell, S. G., Subrath, J. J., Warner, E., Kalra, S., Hunkele, A., Pagirsky, J., Eans, S. O., Medina, J. M., Xu, J., Pan, Y.-X., Borics, A., Pasternak, G. W., McLaughlin, J. P., & Majumdar, S. (2016). Mitragynine/Corynantheidine Pseudoindoxyls As Opioid Analgesics with Mu Agonism and Delta Antagonism, Which Do Not Recruit β-Arrestin-2. Journal of Medicinal Chemistry, 59(18), 8381–8397. https://doi.org/10.1021/acs.jmedchem.6b00748
- Damodaran, T., Chear, N. J.-Y., Murugaiyah, V., Mordi, M. N., & Ramanathan, S. (2021). Comparative Toxicity Assessment of Kratom Decoction, Mitragynine and Speciociliatine Versus Morphine on Zebrafish (Danio rerio) Embryos. Frontiers in Pharmacology, 12, 714918. https://doi.org/10.3389/fphar.2021.714918